In the face of one of the most pressing challenges of our time — the transition to sustainable, fossil-free energy systems — Germany continues to emit greenhouse gases amounting to 750 milllion tons of CO2-equivalent [7]. The energy sector alone is responsible for a third of this. Just 50% of the generated electricity comes from renewable sources, while the situation is more precarious for heat generation with only 17%. Technological, economic, political, and societal solutions are needed for transforming the system.
Our focus is on supporting politics and industry in their transformation efforts for the benefit of society. In doing so, we need to analyze the variety of potential solutions and identify the most promising ways to accelerate the transformation process. Our methods for finding such solutions are based on mathematics and artificial intelligence and identify optimal transformation pathways for multi-sector energy systems from a single neighborhood to the whole of Europe [10].
In the past, the primary focus in planning and operating energy systems has solely been on economic goals, such as minimizing costs while maximizing revenues. However, the ongoing transformation necessitates a shift toward incorporating ecological objectives, like reducing CO2 emissions, and societal considerations, such as minimizing public opposition, simultaneously.
This results in an optimization problem with multiple conflicting targets. For example, the team of the Research Campus MODAL at ZIB has introduced a novel method employing for determining optimal wind farm locations where the desired solutions provide an optimal compromise between the federal targets for wind energy expansion, the availability of potential grid connection points, and the categorization for interventions in the landscape. In addition to the novel modelling approaches, advancements in mathematical solvers at ZIB [6] have made it possible to find these solutions.
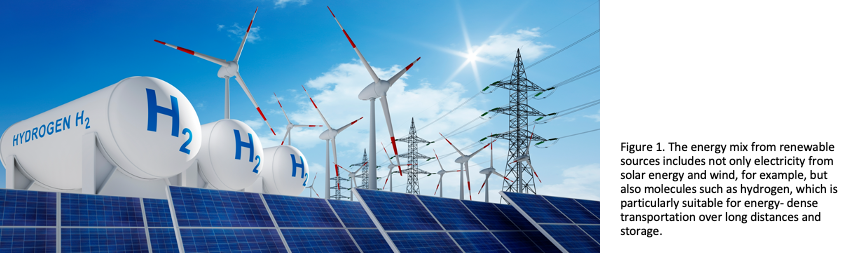
Another example is the optimal investment planning for the Berlin district heating network, which is considered one of the most complex in Western Europe. The objective was to consider CO2 emissions in addition to economic costs. The predominant challenge facing this network lies in its existing centralized structure, characterized by a limited number of large fossil-fired power plants and a scarcity of small combined heat and power plants. The transformative strategy entails a shift towards decentralization, incorpo-rating a greater number of smaller production units. Examples include large heat pumps har-nessing industrial and waste water heat, and geothermal reservoirs. The redesigned system promises enhanced flexibility in unit commitment during operations. However, this requires multiobjective optimization to identify the optimal tradeoff between economical and ecological goals. We devised new methodologies to address such problems, involving millions of variables. The solutions minimize costs and CO2 emissions while maximizing efficiency.
Furthermore, methods developed at ZIB, together with MODAL and the Cluster of Excellence MATH+, allow the dynamical behavior of large energy networks to be predicted in order to steer them optimally, especially regarding the co-transport of green hydrogen [3]. For example, one of the largest gas transmission system operators employs them to forecast the expected hourly gas flows in a large part of Germany [4, 5]. These methods will now be extended to the entire German natural gas market area.
In the future, we want to extend our methods to large consumers and their energy processes. This will enable us to optimize not only energy generation and its transport including large consumers. This holistic optimization allows the costs to be fairly distributed between producers and consumers, based on the advanced mathematical methods we are developing in close cooperation with industry and research partners.
References:
[1] F. Hennings, M. Petkovic, and T. Streubel. On the numerical treatment of interlaced target values - modeling, optimization and simulation of regulating valves in gas networks. Optimization and Engineering (2023). DOI: 10.1007/s11081-023-09812-0.
[2] T. T. Le, Y. Fukumoto, and T. Koch. Linear stability of a simple shear layer between two parallel streams in a shallow water flow. Physics Letters A 493 (2023). DOI: 10.1016/j.physleta.2023.129264.
[3] J. Pedersen, K. Spreckelsen, U. Gotzes, J. Zittel, and T. Koch. Beimischung von Wasserstoff zum Erdgas: Eine Kapazitätsstudie des deutschen Gasnetzes. gwf Gas + Energie (2023).
[4] M. Petkovic, Y. Chen, I. Gamrath, U. Gotzes, N. S. Hadjidimitrou, J. Zittel, X. Xu, and T. Koch. A hybrid approach for high precision prediction of gas flows. Energy Systems 13 (2022), 383–408. DOI: 10.1007/s12667-021-00466-4.
[5] M. Petkovic and J. Zittel. Forecasting and modeling the dynamics of large-scale energy networks under the supply and demand balance constraint. In: AIRO Springer Series: International Conference on Optimization and Decision Science. accepted for publication. 2023.
[6] D. Rehfeldt and T. Koch. Implications, conflicts, and reductions for steiner trees. Mathematical Programming 197 (2023), 903–966. DOI: 10.1007/s10107-021-01757-5
[7] Umweltbundesamt. Emissionsquellen. 2021. URL: https://www.umweltbundesamt.de/themen/klima-energie/treibhausgas-emissionen/emissionsquellen#energie-stationar.
[8] R. Yokoyama, Y. Shinano, and T. Wakui. An effective approach for deriving and evaluating approximate optimal design solutions of energy supply systems by time series aggregation. Frontiers in Energy Re-search 11 (2023), 1–14. DOI: 10.3389/fenrg.2023. 1128681.
[9] I. Yueksel-Erguen, T. Koch, and J. Zittel. Consistent flow scenario generation based on open data for operational analysis of european gas transport networks. In: Operations Research Proceedings. accepted for publication. 2023.
[10] I. Yueksel-Erguen, D. Most, L. Wyrwoll, C. Schmitt, and J. Zittel. Modeling the transition of the multi-modal pan-european energy system including an integrated analysis of electricity and gas transport. Energy Systems (2023). epub ahead of print. DOI: 10.1007/s12667-023-00637-5.