Enabling technologies for complex therapies.
In today’s medicine therapeutic interventions are getting increasingly complex. Functional and physiological data need to be monitored, structures of risk are to be considered, and a lot of technology is involved. In order to master the complexity and to deliver successful treatments, such therapies need to be carefully planned, taking individual anatomy and pyhsiological processes into account. We develop algorithms and demonstrators supporting computer-assisted, model-guided therapy planning.
Orthopedic surgery
Orthopedic surgery is concerned with the treatment of pathologies in shape or function of the musculoskeletal system that may be caused by trauma, degenerative or congenital diseases. Therapy planning in orthopedics comprises the identification of pathological situations as well as the design of therapeutic concepts under consideration of functional rehabilitation. Based on patient specific anatomical models, for instance, pose and shape of anatomical structures can be analysed and both can be compared to nonpathological findings. Using kinematic models also functional aspects can be analyzed, e.g., the range of motion of joints (FN:S. Saevarsson, G. Sharma, H. Ramm, R. Lieck, C. Hutchison, J. Werle, S. Montgomery, C. Romeo, S. Zachow, C. Anglin {2013}. Kinematic differences between gender specific and traditional knee implants. The Journal of Arthroplasty, 28{9}:1543 – 1550.) . Stresses within anatomical structures can be simulated under particular loading conditions, and parts of the anatomical structures can be virtually removed and replaced by implants in order to analyze the performance of the planned configuration (FN:F. Galloway, M. Kahnt, H. Ramm, P. Worsley, S. Zachow, P. Nair, M. Taylor {2013}. A large scale finite element study of a cementless osseointegrated tibial tray. Journal of Biomechanics, 46{11}:1900 – 1906.). With the help of computer-assisted and model-guided therapy planning, different strategies can be preoperatively assessed and the most promising approach can be chosen for implementation. We are developing techniques for 3D shape analysis and establishing 3D shape databases representing various anatomical structures that can be stratified according to gender, age, body weight, and so forth. Within the stratified groups the range of variation of properties like shape or mineral density can be analyzed. An individual patient’s anatomy can then be matched against the shape database in order to assess its pathological state and to determine how to surgically correct skeletal structures, mechanical axis or joint contact regions. In addition, optimally fitting implants can be selected from a set of different types or manufacturers, or even new implant types can be designed that either fit an individual patient or a particular population, whose anatomical average shape can be derived from the database (individual vs. mass customization).
Individual anatomy reconstruction of the knee bones allows 3D planning of implant positioning. Mechanical simulations taking cartilage, patella, tendons, and ligaments into account improve the understanding of degenerative diseases and the functionally appropriate positioning of implants.
Knee implant wear testing is a time consuming process. Efficient simulation of wear can speed up the design process significantly.
Facial Surgery
Preoperative planning of plastic and reconstructive surgery of the head, not only involves functional but also aesthetic aspects. This is especially the case for congenital malformations, where a face or the shape of the head will be heavily altered without having an individual reference. Either rearrangements of bony structures need to be planned, or implants need to be carefully designed to model a new face or head. In both cases the facial appearance needs to be taken into account in order to achieve the best possible aesthetic result under consideration of functional rehabilitation (FN:M. Zinser, S. Zachow, H. Sailer {2013}. Bimaxillary “rotation advancement” procedur es in patients with obstructive sleep apnea: A 3- dimensional airway analysis of morphological changes. International Journal of Oral & Maxillofacial Surgery, 42{5}:569 – 578.). We are working on software tools to perform interactive 3D planning of bone cuts and rearrangements based on digital anatomy as well as to design implant shapes. In addition, we work on models and algorithms to realistically simulate mechanical behaviour of biological tissues. We also develop methods to establish a database of 3D face models that can be used to derive an objective for facial reconstruction. Furthermore, we develop methods to determine the shape of implants for an individual patient with regard to a desired facial appearance. That way surgeons can directly shape a face, automatically receiving a blueprint for implants that once inserted lead to the planned aesthetic outcome.
Augmentation implants replace traumatized bone or fill voids left by malformed bones. An important aspect is to restore a socially accepted facial shape, for which the appropriate implant shape has to be determined.
In a Nutshell
- Modern interventions are increasingly complex.
- Many medical areas are affected: osteotomic surgery, facial surgery, implant design, cardiac diseases, and more.
- Patient-specific anatomical models and simulations thereon are necessary for improved reliability of treatment decisions.
Cardiovascular Diseases
Changes in transmembrane voltage induce calcium release and myocard contraction. Simulation of the excitation fosters understanding of fibrillation onset and stability of spiral wave patterns, improving individual therapy planning.
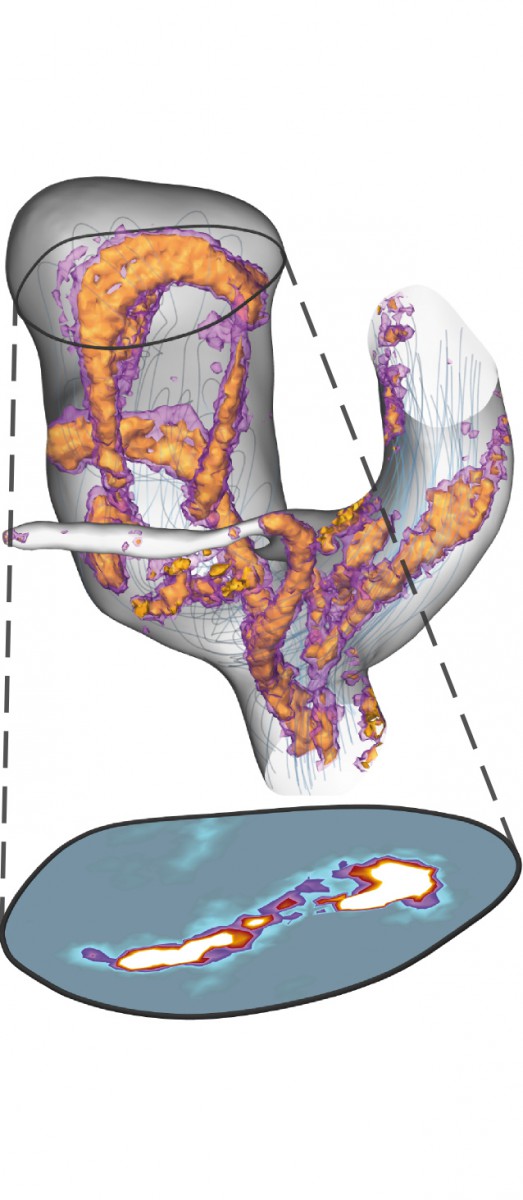
Probability density of vortex cores in an aneurysm.
Treatment decisions for cardiovasclar diseases, e.g., in valve replacement, resynchronization therapy, or ablation therapy, should be based on the benefit of the patient. Large inter-individual variations of the outcome make the prediction of the benefit difficult, e.g., some patients develop a ventricular hypertrophy after valve replacement, and some do not. Some aneurysms remain the same size over years, and some grow quickly with the danger of rupture. A reliable patient-specific simulation of the relevant physiological processes is often limited by the sheer complexity of the cardiovascular system, involving different scales from mitochondrial metabolism to systemic circulation and effects from ion transport in myocytes to fluid dynamics. Therefore, individual therapy planning is still far from clinical routine. We are working on efficient simulation methods for the electrical excitation of the heart and the induced mechanical contraction. This includes not only adaptive, error controlled solvers for partial differential equations, but also data compression algorithms for the efficient storage of simulation results for optimization purposes. E.g., implantable cardioverter-defibrillators can detect the onset of life-threatening ventricular fibrillation and terminate this condition by applying a sufficiently strong electrical impulse to the myocard. “Pushing this big reset button of the heart is painful and shocking for the patient”, explains PhD student Sebastian Götschel. “One goal in designing the pulse shape is to minimize its amplitude while maintaining the effectivity”.
Estimating the rupture risk of aneurysms is important to decide whether to perform surgery or not. This risk depends on the flow patterns of blood in the aneurysm and the resulting wall shear stresses. The stochastic nature of these quantities makes a reliable interpretation difficult. Uncertainty visualization methods can help to understand the risk factors better.
Medical Image and Geometry Processing
Computer-assisted therapy planning in medicine is heavily based on medical imaging technology. Therefore, image data are not only to be visualized but often also to be converted into individual 3D patient models, sub-divided into various anatomical structures of interest (FN:S. Zachow, M. Zilske, H.-C. Hege {2007}. 3d reconstruction of individual anatomy from medical image data: Segmentation and geometry processing. ZIB Report 07-41, Zuse Institute Berlin, Takustr.7, 14195 Berlin.). In order to faithfully extract anatomical 3D models from medical image data, we are developing algorithms for image enhancement, segmentation, and geometry reconstruction. Converting segmented image data into 3D surfaces or volumetric grids makes them accessible to computational methods for mathematical analysis and simulation. In addition, interactive visualization methods are developed for presenting image and simulation data to the domain experts in an intelligible manner and software prototypes to our clinical research collaborators.
Comparing projections of shape models with actual X-ray images allows to estimate individual 3D geometry from few X-ray images, reducing the radiation dose compared to CT scans.
Medical Geometry Processing
Though medical image data can be directly visualized for diagnostic purposes, interactive, model-guided planning and simulation often requires three-dimensional geometric models representing individual patients’ anatomy. Therefore, we are developing algorithms for the conversion of segmented image data into geometrical representations like surface meshes or volumetric grids, making them accessible to computational methods for interactive therapeutic modifications and numerical simulations employing, for instance, advanced finite-element techniques.
We are also developing methods to establish and to improve statistical shape models. One important step in building shape models is the registration of complex shapes onto an average. Among others, we are investigating large deformation diffeomorphic mapping methodsthat use a deformation of a spatial embedding for the registration of shapes. Decoupling shape representation and space deformation allows a better adaptation of the discretizations to the solution. Statistical shape models are the basis for the generation of new anatomical atlases that not only show averaged shapes of anatomical structures but also the variation in shape of such structures. The latter is important for morphological analysis in general, discrimination of pathologies, classification, and even the human-centered design of implants or prostheses. To understand complex relationships between shape and other factors or categorial information, we are developing methods to visually explore and to analyze shape models.
Image Processing
We are developing efficient and robust algorithms for medical image segmentation deploying so called shape priors. Such shape priors represent averaged 3D shapes of many similar anatomical structures, implicitly describing the full range of variation in shape that typically exist in any population. For fully automated segmentation of arbitrary medical image data, like computed tomography, magnetic resonance imaging, or ultrasound, shape priors are enriched with modality specific image information. Within an optimization scheme these shape and appearance models are plausibly deformed until shape and image features possibly coincide (FN:D. Kainmüller, H. Lamecker, M. Heller, B. Weber, H.- C. Hege, S. Zachow {2013}. Omnidirectional displacements for deformable surfaces. 17{4}:429 – 441.). Our algorithms have been successfully applied for fully automatic segmentation of a broad range of different anatomical structures from different imaging modalities. In addition, highly parallelized image filtering, registration, and segmentation algorithms are being developed – deploying modern hardware architectures to handle an ever increasing amount of medical image data in reasonable time (FN:J. Lamas-Rodríguez, D. Blanco Heras, F. Argüello, D. Kainmueller, S. Zachow, M. Boo {2013}. Gpu-accelerated level-setsegmentation. Journal of Real-Time Image Processing, pages 1 – 15. epub ahead of print.) or to solve complex 3D reconstruction problems. Moritz Ehlke, PhD student in the Berlin School of Regenerative Therapies explains his work (FN:M. Ehlke, H. Ramm, H. Lamecker, H.-C. Hege, S. Zachow {2013}. Fast generation of virtual x-ray images for reconstruction of 3d anatomy. Visualization and Computer Graphics, IEEE Transactions on {Volume: 19, Issue: 12} pp. 2673 - 2682, 19{12}.): “Reconstructing 3D anatomies from few projective X-ray images is only possible with very good knowledge of the arising shapes, as it is encoded in shape priors. Still, the necessary simulation of X-ray projections requires the computing power of modern GPUs.”
Numerical Methods for Biomedical PDES
The physical and physiological processes that are relevant in complex interventions, such as electrical excitation, heat transport, or mechanical behaviour of soft tissues, bones and implants, are usually described by partial differential equations (PDEs). In order to be able to predict the impact of treatments and to optimize their parameters, we develop finite element methods and optimization algorithms. One focus of our research are adaptive, error-controlled methods combining reliability and efficiency.
S. Götschel works on trajectory compression for finite element computations.
Time Integration
Dynamic problems such as cardiac excitation or implant wear simulation require a time stepping scheme that integrates well with adaptive spatial finite element discretization. Recently we turned to spectral deferred correction (SDC) methods, which are simply structured iterative methods for solving implicit collocation systems. Interleaving SDC iterations and mesh refinement, as well as amortizing the overhead of adaptivity over longer time steps possible with higher-order methods, bear the promise of improving overall efficiency. The deteriorating convergence rate of SDC methods on nonequidistant time grids can be accelerated significantly by a special construction of the SDC sweeps (FN:M. Weiser {2013}. Faster SDC convergence on non-equidistant grids with DIRK sweeps. ZIB Report 13-30.).
Trajectory Compression
In time-dependent 3D optimization problems, such as optimal control of implantable cardioverter-defibrillators or shape optimization of knee joint prosthesis for minimal wear, adjoint gradient computation for fast minimization requires the repeated storage of a full 4D simulation for adjoint gradient computation. This can impose a significant difficulty in terms of both, storage capacity and storage bandwidth. In a Matheon project we have developed lossy compression of finite element solutions on adaptively refined meshes, reducing the memory demand as well as the memory bandwidth by a factor of more than 20 without impeding the convergence of the optimization algorithm (FN:S. Götschel, C. von Tycowicz, K. Polthier, M. Weiser {2013}. Reducing memory requirements in scientic computing and optimal control. ZIB Report 13-64.), (FN:S. Götschel, M. Weiser {2013}. Lossy compression for PDE-constrained optimization: Adaptive error control. ZIB Report 13-27.). “It’s similar to MPEG video compression, just that it works on unstructured grids instead of on pixels, and respects the error transport through the adjoint equation rather than visual artifact perception”, says PhD student S. Götschel.
Optimal Control
Complex therapies often exhibit a moderate to large number of parameters to fix, e.g., the shape of augmentation implants or the positioning of joint implants. Choosing parameters for an optimal outcome can be rather challenging. We work on optimization algorithms suggesting optimized therapy parameters. Sometimes this involves basic research: “Very little is known about the structure of nonlinear elasticity solutions’’, PhD student Lars Lubkoll says, “so we are glad we could prove the existence of minimizers” (FN:L. Lubkoll, A. Schiela, M. Weiser {2012}. An optimal control problem in polyconvex hyperelasticity. ZIB-Report 12-08.).
Software Prototypes for Therapy Planning
Algorithmic developments have to be implemented as a prototype software to be evaluated in clinical research. For the construction of such demonstrators we make use of the Amira and Kaskade 7 software developed at ZIB.
Software prototype for interactive patient-specific planning of bone anchored hearing aids. Research cooperation with 1000shapes GmbH and Unfallkrankenhaus Berlin-Marzahn (UKB).
In a Nutshell
We establish demonstrators of complete software pipelines covering medical image data processing, geometry reconstruction, biomedical simulation and therapy planning. Numerical developments focus on adaptive solvers for PDEs describing biomechanics or physiological processes and optimization techniques.
Finite Element Toolbox Kaskade 7
The workhorse for PDE problems is our finite element toolbox Kaskade 7 based on the DUNE libraries. Its C++ template structure allows a flexible, typesafe, and efficient mix-and-match approach to arbitrarily discretized systems of PDEs. The toolbox has been used to solve problems from various medical areas including cardiac excitation, hyperthermia cancer treatment planning, soft tissue deformation, and implant design. Recent improvements comprise spectral deferred correction methods for time integration, discontinuous Galerkin methods, and better memory access patterns on NUMA machines.
Visualization Framework AMIRA
Amira is a visualization and data analysis software that was designed and developed at ZIB. It is now commercially developed and distributed under the product names Amira and Avizo by FEI Visualization Sciences Group. ZIB Amira is a variant of Amira that ZIB uses for ongoing research with cooperation partners. It contains well-known visualization and data analysis methods and results of ongoing research in various fields, such as image analysis, geometry processing, flow analysis, and molecular visualization. Prototypes of interactive therapy planning tools are implemented as special purpose modules in Amira.